
Expired activity
Please go to the PowerPak
homepage and select a course.
Core Competencies in Health-System Pharmacy: Pediatric Pharmacy Practice
Introduction
“Children are NOT little adults.”
While most people consider pediatrics as a single specialty, this specialty, in fact, consists of a diverse population. From extremely-low-birth-weight premature neonates weighing as little as 0.5 kg, to full term neonates, infants, children, to adolescents who may weigh more than 100 kg, each of these subgroups can exhibit different responses to medications, doses, and inactive ingredients, depending on the pharmacokinetic and pharmacodynamic variations. The lack of commercially available dosage forms and concentrations appropriate for the various subgroups of pediatric patients present additional challenges when caring for this vulnerable population.
In this program, the major differences in absorption, distribution, metabolism, and elimination of medications in the pediatric population are reviewed and the clinical implications outlined. Important considerations when administering medications to pediatric patients are discussed. Lastly, the importance of all pharmacists helping to safeguard the medication-use process to protect this very vulnerable patient population is highlighted.
DEFINITION
For discussing development and medication use in pediatric patients, terms must be used carefully and appropriately. Depending on their gestational age at birth, pediatric patients have important differences that affect drug metabolism and other clinically important factors both during development and in some cases, into adulthood.
Important terms to describe development as used in pediatric practice are presented in Table 1.
Table 1. Definitions of Terminology Used in Pediatric Practice |
Terms |
definition |
Gestational age (GA) |
Time from conception to birth |
Postnatal age |
Chronological age since birth |
Premature neonate |
Neonate born before 37-week GA |
Full-term neonate |
Neonate born at or after 37-week GA |
Neonate |
Birth to <28 days (4 weeks) |
Infant |
28 days to <12 months old |
Toddler |
1–2 years old |
Child |
1–12 years old |
Adolescent |
13–18 years old |
Adult |
>18 years old |
Source: Reference 1.
Abbreviations used: GA, gestational age. |
DEVELOPMENTAL PHARMACOLOGY
Understanding drug disposition during the various development stages of maturation will help one recognize why “children are NOT little adults.” Appropriate application of this knowledge is the reason why Young’s rule or Clark’s rule, which extrapolate a proportion of an adult dose for a pediatric patient based on the weight of the patient, is no longer a standard of practice. In fact, this practice has resulted in harm to patients since pediatric patients; neonatal patients in particular metabolize certain drugs very differently than adults and may exhibit adverse reactions not seen in adults.
Absorption
Gastrointestinal Tract
Intraluminal pH affects ionization of drugs and hence the amount of drug being absorbed through the gastrointestinal (GI) tract. At birth, gastric pH of a neonate is neutral, reflective of the pH of amniotic fluid. The gastric pH drops to 2 to 3 within hours after birth, returns to less than 4 at around day 10 of life and reaches adult level by age 2 to 3.
The elevated gastric pH early in life results from lower gastric acid secretion. The implication for this difference is that drugs that are weak bases have increased absorption, while weak acids such as phenobarbital and phenytoin have decreased absorption and hence require higher doses to achieve therapeutic drug levels. 2, 3 In addition, the bioavailability of acid-labile drugs such as penicillin is increased since they are not broken down in the higher gastric pH.1
Gastric emptying is delayed in neonates, and the age at which they reach adult levels varies depending on the degree of prematurity, type of feedings, and presence of other conditions. By 6 to 8 months of age, the infant has an adult rate of gastric emptying.1 This physiologic factor explains the “wet burps” neonates and young infants often experience after feedings. Delayed gastric emptying can also result in increased absorption of drugs that are absorbed in the stomach since medications have a longer “dwell time” in the gastric cavity. On the other hand, delayed absorption, hence delayed onset of action, of drugs absorbed in the small intestine should be expected. This is most important for drugs such as sedatives or analgesics/antipyretics, for which a fast onset of action is expected. Clinicians should administer oral sedatives at a minimum of 30 minutes to an hour before procedures in neonates and young infants to allow adequate time for the drug to be absorbed. Similarly, repeat sedative doses should not be administered until 30 minutes to an hour after the first dose to allow for adequate time for absorption and avoid excessive or extended sedation.
Activity of intraluminal bile acids is also decreased at birth and throughout the first 6 months of life, resulting in reduction in absorption of lipid-soluble drugs.1
Percutaneous Administration
The rate of percutaneous absorption is inversely related to stratum corneum thickness and directly related to skin hydration. Decreased stratum corneum thickness in neonates and increased skin hydration increase percutaneous absorption of substances that otherwise may not be absorbed in adults and thereby increase the potential for adverse reactions. The stratum corneum, however, is fully mature in full-term and preterm neonates at 2 weeks of life.1–3 The surface area to body weight ratio is also higher in neonates and children compared with adults; equal application per area of skin results in greater dose absorbed systemically based on body weight.1–3 These are all reasons why sunscreen is generally not recommended for infants younger than 6 months of age.4
Other common topical agents pharmacists should be mindful of when counseling parents of neonates and young infants include topical steroids and topical antibiotics. Excessive use of topical steroids may lead to adrenal suppression and other systemic adverse reactions in neonates.5 When a topical steroid is required, a product with the lowest effective potency should be selected. In addition, parents should be counseled on using it sparingly, especially around highly vascularized areas such as the diaper area, and on using it on an as-needed basis if possible. Topical antibiotics containing neomycin (e.g., bacitracin zinc, neomycin sulfate, polymyxin B sulfate) should be avoided, instead non-neomycin-containing topical antibiotics (e.g., bacitracin zinc and polymyxin B sulfate) should be recommended for neonates. This is because neomycin causes the highest incidence of nephrotoxicity and ototoxicity — and specifically cochlear toxicity — among all aminoglycosides when absorbed systemically (hence it is not available as an injectable formulation).6 In addition, at high serum levels, polymyxin B sulfate may cause neurotoxicity and nephrotoxicity and bacitracin may cause nephrotoxicity.7,8 Therefore, topical antibiotics with these 2 active ingredients should be applied sparingly as well.
In the acute care setting, alcohol and povidone iodine are frequently used as antiseptics. In one case report, a 27-week preterm infant had very high ethanol level after 95% ethanol was used for umbilical catherization. Hypothyroidism has been reported in neonates, especially those born prematurely, after repeated and prolonged use of povidone–iodine as an antiseptic.9 In practice, alcohol wipes are used sparingly as an antiseptic in preterm and full-term neonates, and povidone–iodine should be used with caution in preterm neonates.
Before applying topical agents to neonates and infants younger than 6 months of age, potential systemic toxicity if absorbed should be investigated before proceeding. In addition, use of transdermal patches below the U.S. Food and Drug Administration (FDA)–approved lower age limit should be avoided since the dose delivered by the patch may not be appropriate for younger children.
Intramuscular Administration
Neonates and young infants have reduced muscle mass and blood flow to muscles and inefficient muscle contractions. Combined with specific characteristics of drugs, these factors result in variable, delayed, or decreased absorption of drugs administered via intramuscular route.2,3 In addition, the maximum volume for each intramuscular injection based on the age of the pediatric patient may necessitate multiple injections (see Table 2), making this a less desirable route of administration.
Table 2. Maximum Volume per Intramuscular Injection Site in Pediatric Patients |
|
Neonate & Infant |
Toddler |
Child |
Adult |
Maximum Volume per Site |
0.5 mL |
1 mL |
2 mL |
5 mL |
Source: Reference 3. |
Rectal Administration
Large variations in rectal pH and blood flow to the rectum, together with the greater propensity for infants and children to expel solid drugs from their rectum result, in erratic absorption of drugs such as acetaminophen.2,3
Distribution
Total body water is about 80% of total body weight in neonates, much lower than the 55% to 60% in adults. Total body water gradually decreases with age while total body fat increases from 10%–15% to 15%–30%. This change in body composition has a direct impact on drug distribution.
Hydrophilic drugs, such as aminoglycosides, have increased volume of distribution and hence require a higher dose per weight to reach therapeutic concentration in neonates. The volume of distribution and corresponding dose of hydrophilic drugs gradually decreases with age (see Table 3). On the contrary, lipophilic drugs, such as diazepam, have a lower volume of distribution, thus requiring a lower dose per weight in neonates to reach an equivalent therapeutic level compared to adults.2,3
Table 3. Volume of Distribution and Half-Life with Corresponding Conventional Dose and Frequency of Gentamicin by Age Group |
Age Group |
Volume of Distribution (L/kg) |
Dose (mg/kg/dose) |
Half-Life (h) |
Dosing Frequency |
Neonates |
0.45 |
4–5 |
3-11.5 |
Every 24–48 hours, dependent on gestational and postnatal age |
Infants |
0.4 |
2–2.5 |
4 |
Every 8 hours |
Children |
0.35 |
2–2.5 |
2 |
Every 8 hours |
Adolescents |
0.3 |
2–2.5 |
1.5 |
Every 8 hours |
Adults |
0.2–0.3 |
1–1.7 |
2 |
Every 8 hours |
Source: Reference 10. |
Neonates and infants have decreased plasma protein concentrations, such as albumin and alpha-1 acid glycoprotein, as well as decreased protein-binding capacity due to presence of fetal albumin. In addition, competition for binding sites by endogenous compounds such as bilirubin further displaces drugs from their protein-binding sites. All of these factors contribute to a larger apparent volume of distribution and increased free (unbound) drug concentrations. Since free drugs are the therapeutically active drugs, increased free drug concentrations will increase therapeutic effect or toxicity.
The impact of these physiologic differences in neonates and infants is most pronounced for drugs that are highly protein bound.2,3 For example, when administering phenytoin or fosphenytoin to neonates, it is important to obtain a free plasma phenytoin concentration instead of the usual total plasma phenytoin concentration. A total plasma phenytoin concentration that is within normal range may result in a free concentration that is above therapeutic range because of decreased protein binding of phenytoin. Medications such as ceftriaxone and sulfonamides (e.g., sulfamethoxazole/trimethoprim) can also displace endogenous bilirubin from its binding site, increasing free (unbound) bilirubin concentration that can more easily cross the blood brain barrier and cause kernicterus (characterized by lethargy, poor feeding, hypotonia, dystonia, seizures, hearing loss, developmental delayed and death). That is why ceftriaxone and sulfonamides are usually avoided during the first 1–2 months of life.
Metabolism
Phase I Reactions
Phase I reactions — which include oxidation, reduction, and hydrolysis — are primarily performed by the cytochrome P450 (CYP) enzyme system. Maturation of this system varies in neonates and infants; some isoenzymes are active in utero while others do not exhibit activity until a few months after birth. Generally, adult enzyme activity levels are reached by 2 years of age, but some activity during childhood can exceed that of adults. See Table 4 for a summary of CYP enzyme activities maturation and their effect on drugs.
At birth, CYP3A7 is the predominant CYP isoenzyme responsible for metabolism of many drugs, but its activity promptly declines thereafter, having minimal activity after the first month of life and undetectable in adults. On the other hand, CYP3A4 and CYP3A5 both have very low activity at birth but rapidly increases during the first month of life and takes over metabolism of drugs as CPY3A7 activity wanes. CYP2E1 activity rapidly rises within hours after birth, followed by CYP2C9, CPY2C19, and CPY2D6. Lastly, CYP1A2 activity appears at 1 to 3 months of life.2,3,11
Table 4. Cytochrome P450 Enzymes Activities Maturation and Effect on Drugs2,3,11 |
CYP Enzymes |
Maturation of Enzyme Activities |
Drugs Affected |
CYP3A7 |
Activity presents at birth, rapidly declines in first month of life and undetectable in adults |
Many drugs |
CYP3A4 |
Activity appears during first week of life, rapidly rises during the first month of life |
Many drugs |
CYP3A5 |
Activity appears during first week of life, rapidly rises during the first month of life |
Many drugs |
CYP2E1 |
Activity rapidly rises within hours after birth and in the first month of life, reaches adult level after 1 year |
Acetaminophen, phenobarbital, anesthetics, ethanol, nicotine |
CYP2C9 |
Activity appears during first week of life, exceeds adult activity during childhood, then declines to adult activity level |
Nonsteroidal anti-inflammatory drugs, phenytoin |
CYP2C19 |
Activity appears during first week of life, rapidly increase thereafter |
Antiepileptics, proton pump inhibitors |
CYP2D6 |
Activity rapidly rises around 2 weeks of life, matures by 10 years old |
Many drugs, codeine |
CYP1A2 |
Activity appears at 1–3 months of life, gradually increases in infancy, exceeds adult activity during childhood, then declines to adult activity level |
Methylxanthines |
Cisapride, a prokinetic agent that is primarily metabolized by CYP3A4, was widely administered to preterm and full-term neonates for gastroesophageal reflux after its FDA approval in July 1993. Numerous clinical studies have demonstrated clinical efficacy of cisapride in neonates and infants, and all reported minor adverse reactions including diarrhea, abdominal pain, nausea, vomiting, headache, and constipation. However, reports of tachyarrhythmia, torsades de pointes, and eventually QT interval prolongation and death were subsequently reported in adults. This was followed by reports that accumulation of cisapride, especially in premature neonates, could result in QT prolongation and death, which were previously misdiagnosed as sudden infant death.12 The drug was consequently removed from the U.S. market July 2000.
Cisapride is a prime example that underscores the importance of understanding developmental pharmacology and pharmacokinetics of drugs in neonates and infants. A review of literature found no pharmacokinetics studies on cisapride conducted in neonates and infants prior to 2000. While other factors such as high doses used and concomitant administration with drugs that inhibit CYP3A4, such as fluconazole and erythromycin, increased plasma levels of cisapride and resultiedin QT prolongation and death, use of this drug in preterm and full-term neonates who had immature CYP3A4 activity further exacerbated the problem.
Theophylline demonstrated an entirely different metabolic pathway in premature neonates compared with adults. Theophylline is metabolized by methylation (reduction reaction) in premature infants, producing caffeine; in adults, caffeine is demethylated (oxidation reaction) to theophylline.13
Phase II Reactions
Phase II reactions or conjugation reactions include glucuronidation, sulfation, methylation, and acetylation. Maturation of these conjugation pathways occurs at different times. Sulfation activity is well developed in neonates. Glucuronidation and acetylation activities are both low at birth and continue to develop until reaching adult levels at 3 to 4 years of life.11
Chloramphenicol is metabolized by the glucuronidation pathway, which is very immature in neonates. Administration of chloramphenicol to neonates have resulted in accumulation of the drug and gray baby syndrome, which is characterized by circulatory collapse and death.3
Acetaminophen, which is known to be metabolized through the glucuronidation pathway, can be safely administered to neonates and infants. This is because in neonates, acetaminophen is preferentially metabolized through the sulfation pathway, which is well developed at birth. As the glucuronidation pathway matures, the drug is increasingly metabolized through the glucuronidation pathway and sulfation decreases.14 These two examples further demonstrate that “children are NOT little adults,” as they do not process drugs the same way as adults.
Elimination
Drugs are eliminated by the kidneys via glomerular filtration, tubular secretion and tubular reabsorption. While nephrogenesis is complete by 36-week gestation, glomerular filtration rate (GFR) is very low at birth (2–4 mL/minute/1.73 m2). In full-term neonates, GFR increases rapidly during the first 2 weeks of life, reaches half the adult values by 3 months of age, increases to adult values by 8 to 12 months of age, increases above adult values during childhood, and then decreases back to adult values.
At birth, GFR is even lower in preterm neonates; development is slower than in full-term neonates during the first week of life and reaches full-term level at 4 to 5 weeks of life. Consequently, dosing frequency of drugs that are primarily eliminated through GFR is adjusted accordingly based on gestation age and chronological age of the neonate (see Table 3).
Tubular secretion is immature at birth and reaches adult capacity during the first year of life. Weak acids such as penicillin, sulfonamides, and cephalosporins are poorly secreted, thus prolonging their half-lives in neonates and infants. Lastly, tubular reabsorption is also decreased and improves to adult level during the first year of life.2,3,11
Equations to estimate GFR in pediatric and neonatal patients are different from those used for adults. Since 2009, the updated Schwartz equation, commonly known as Bedside Schwartz equation is used to estimate GFR in pediatric patients 1–18 years of age. For neonates and infants younger than 1 year of age, the Brion equation should be used.15
Bedside Schwartz equation:
eGFR = 0.413 x Ht/Scr
Brion equation:
eGFR = k x Ht/Scr
where k = 0.33 for preterm infants
k = 0.45 for full term infants
eGFR = estimated glomerular filtration rate in mL/min/1.73 m2
Ht = Height in cm
Scr = serum creatinine in mg/dL
Case Studies on Developmental Pharmacology
Chlorhexidine
Is 2% chlorhexidine safe for use topically as an antiseptic in preterm and full-term neonates to prep for peripheral and central catheter insertion and routine care of catheter sites?
The following information is available on Micromedex: ®, “Absorption: Topical: Little or no absorption occurs through intact skin of adults [referenced to product information for Hibiclens®]. Low levels of chlorhexidine were found in heel prick blood samples of 10 infants and in venous blood samples of 5 infants who had been bathed in full strength Hibiscrub®. However, contamination of the samples may be responsible for the reported levels.” “INGESTION: Ingestion of 150 mL of a 20% chlorhexidine gluconate solution (30 grams of chlorhexidine or 400 mg/kg) resulted in pharyngeal edema, necrotic esophageal lesions, elevated liver enzymes, and diffuse fatty degeneration and lobular necrosis of the liver. Ingestion of 30 mL of a 4% solution did not result in adverse [gastrointestinal] effects. Ingestion of 0.12% mouth rinse is not expected to be irritating. INTRAVENOUS (IV): Inadvertent IV administration of 0.8 gram of 20% chlorhexidine gluconate resulted in hypotension, tachycardia, and acute respiratory distress syndrome. Hemolysis due to hypotonicity has been reported following accidental IV administration of 1 L of 1:5000 chlorhexidine.”16
Evaluation: Since percutaneous absorption is expected in neonates due to increased permeability of their skin, the information about absorption in adults is irrelevant. While low levels of chlorhexidine were found in infants bathed in full-strength Hibiscrub (4%), the amount of 2% chlorhexidine use for prepping of catheter insertion is very much lower; hence the amount of chlorhexidine absorbed systemically is likely negligible. Systemic toxicity is associated with only with exposure to larger amounts of chlorhexidine. Hence, even if low amounts of chlorhexidine is absorbed, these are unlikely to cause systemic toxicity in preterm or full-term neonates.
Recommendation: It is safe to use chlorhexidine 2% topically as an antiseptic in preterm and full-term neonates to prep for peripheral and central catheter insertion and routine care of catheter sites.
Acarbose
A pediatric surgeon writes an order for acarbose 12.5 mg with every meal 3 times a day (half of the initial dose for adult) for a 4-year-old child who is s/p Nissen fundoplication experiencing dumping syndrome. Dumping syndrome is characterized by rapid gastric emptying after meals, resulting in hyperglycemia immediately postprandial and subsequent reactive hypoglycemia, which is most concerning in a young child. Since acarbose lowers postprandial blood glucose concentrations by delaying hydrolysis of complex carbohydrates, thus decreasing the rate of glucose absorption, it could help smooth out the glucose level in this patient with dumping syndrome. What is the best action of the pharmacist to take regarding this order?
The following information is available on Micromedex: “PEDIATRIC DOSING: The safety and efficacy of acarbose are not established in pediatric patients.” “Absorption: Oral: 0.5% to 2%. After acarbose is degraded to its component sugar units by intestinal bacteria, up to 35% of the dose is absorbed. Metabolism: Intestinal wall, extensive.Acarbose is degraded in the intestine by bacterial and digestive enzymes. Glucose units are removed from the acarbose molecule. Metabolites: 4-methylpyrogallol derivatives, inactive. Several metabolites have been identified of which the majority are 4-methylpyrogallol derivatives (i.e., sulfate, methyl, and glucuronide conjugates). One metabolite retains some activity. Excretion: Renal Excretion 2% (parent drug), 34% (inactive metabolites). Feces, 51%.” “TOXICITY: Toxicity following overdose is rare. Overdose effects are anticipated to be an extension of adverse effects following therapeutic use and may include flatulence, diarrhea, and abdominal pain. RARE: Less frequent adverse effects that have occurred include elevated hepatic enzyme concentrations, anemia, ileus, and acute allergic reactions.”17
Evaluation: Systemic absorption of acarbose is negligible in adults. Since this is a 4-year-old child, gastric pH and gastric emptying should be similar to that of an adult, hence systemic absorption should be negligible just as in adults. Even in overdose situation, the adverse effect profile appears to be benign. The potential benefit of acarbose in preventing reactive hypoglycemia appear to outweigh the potential adverse effects, which are relatively benign and can be monitored.
Recommendation: The pharmacist should fill the order and recommend that the patient’s glucose level be monitored closely for efficacy of the drug. In addition, potential adverse reactions should be closely monitored as well.
Pamidronate
A 5-day-old full-term neonate is diagnosed with the most severe form of osteogenesis imperfecta, a genetic disorder that causes the bones to fracture easily. The neonatologist wants to initiate pamidronate 0.5 mg/kg/day IV on day 1, then 1 mg/kg/day on day 2 and 3, and provided the pharmacist with an article that used that dosing regimen in children 2 years and older. The pharmacist reviews the patient’s medical record and notices that the patient had an elevated serum creatinine level since birth and an elevated gentamicin trough concentration 2 days ago. What is the best action of the pharmacist to take regarding this order?
The following information is available on Micromedex: “Metabolism: Pamidronate is not metabolized. Excretion: Renal: 46% unchanged within 120 hours. Patients with renal impairment demonstrated a trend toward a lower percentage of unchanged drug in the urine compared to patients with normal renal function. Renal clearance of pamidronate correlates closely to creatinine clearance. Elimination Half-life: Parent compound: 28 hours.” “Onset: 1 to 2 days. Peak Response: Hypercalcemia of malignancy, IV: 6 days; Paget's disease, IV: 1 to 3 months. Duration: Single Dose:Hypercalcemia of malignancy, IV: 2 weeks to 3 months.” “Overdose of these medications are rare. OVERDOSE: Hypocalcemia has been reported following single dose ingestions of 4000 to 6000 mg etidronate and total doses of 225 to 300 mg pamidronate given IV over 2.5 to 4 days. Fever and hypotension have also been reported with IV administration of 285 mg pamidronate daily for 3 days. In general, overdose effects are anticipated to be an extension of adverse effects at therapeutic doses. ADVERSE EFFECTS: COMMON: Hypertension, injection site reaction, hypocalcemia (up to 17%), hypokalemia (up to 18%), hypomagnesemia (4% to 12%), hypophosphatemia, loss of appetite, nausea, vomiting, anemia, urinary tract infectious disease,cough, dyspnea, fatigue, malaise. Serious: Arthralgia, bone pain, myalgia, osteonecrosis of jaw, seizure, deteriorating renal function, focal segmental glomerulosclerosis, nephrotoxicity.”18
Evaluation: Since pamidronate is administered intravenously, absorption is not a concern. It is not metabolized, and thus immature phase I and II reactions are not of concerns either. However, clearance of pamidronate correlates closely to creatinine clearance. As discussed earlier, neonates are born with very low GFR (2–4 mL/min). Even though it rapidly increases during the first 2 weeks of life, it does not reach adult values until 8–12 months of age.
In particular, this patient’s renal function is worse than a normal 5-day-old full-term neonate as evident by increased serum creatinine and gentamicin trough concentrations. Therefore, the article with the dose used in children 2 years and older is not applicable to this patient. Decreased clearance and potential accumulation of pamidronate is a real concern for this patient.
Coupled with the long duration of action of the drug (weeks to months), these factors could result in prolonged duration of adverse reactions, including electrolyte abnormalities, seizures, and nephrotoxicity. Furthermore, abnormally low plasma calcium, potassium, and magnesium concentrations may result in cardiac rhythm abnormalities. Therefore, the potential risk of pamidronate adverse reactions in this patient is much higher than the potential risk of bone fractures from falls (since it is less likely that a neonate would have a fall than an older infant or toddler).
Recommendation: The pharmacist should advise the neonatologist that based on the high potential risk of pamidronate and the low benefit from the treatment at this point, pamidronate therapy should be ideally delayed until the patient is 8–12 months of age; at the minimum, the drug should not withheld until the patient reaches 3 months of age when the renal function should approximate about half of that in adults.
Day-to-Day Application and Formulary Considerations
When considering a medication for use in adult patients or addition to the institution’s formulary, clinicians consider pharmacokinetics and pharmacodynamics data as well as clinical efficacy and side effects profile of the medication. Once safety and efficacy are established, the cost of the medication may be considered to determine if prescribing restrictions should be applied.
When evaluating a medication for use in pediatric patients, the same adult data should be evaluated. In addition, pediatric clinical trials and ideally, pediatric pharmacokinetics data should be included. If pediatric pharmacokinetics data are not available, the maturation effects on pharmacokinetics of medications (absorption, distribution, metabolism and elimination) should be considered. Ultimately, safety and efficacy of the medication in pediatric patients should be established. The risk versus benefit of the medication should always be taken into consideration.
At times, a more costly medication in the same therapeutic class may be selected if the more costly medication is the only one in the class that has been studied in pediatric patients.
ADMINISTRATION CONSIDERATIONS
Besides developmental pharmacology, certain inactive ingredients and challenges with dosage forms are also important details to consider when administering medications to pediatric patients.
Inactive Ingredients
Benzyl Alcohol
Benzyl alcohol is a common antibacterial agent used as a preservative for injectable medications. In 1982, the FDA, Centers for Disease Control and Prevention, and the American Academy of Pediatrics (AAP) issued a bulletin warning against the use of fluids and diluents containing benzyl alcohol in neonates. Gasping baby syndrome presented as severe progressive metabolic acidosis, respiratory distress with gasping (hence the name of the syndrome), bradycardia, hypotension, cardiovascular collapse, convulsion, intracranial hemorrhage, encephalopathy, and eventually death.
The gasping baby syndrome occurred in 10 preterm neonates each from 2 neonatal intensive care units in the United States and was associated with large cumulative daily doses of benzyl alcohol in bacteriostatic normal saline and heparinized bacteriostatic sodium chloride used to flush central and peripheral catheters and bacteriostatic water used to reconstitute medications. The large amount of benzyl alcohol administered to these neonates coupled with the immature oxidation and conjugation pathways in the liver led to accumulation of benzyl alcohol and its associated toxicity.19,20
A case-control study demonstrated that gasping baby syndrome developed when total daily doses of benzyl alcohol exceeded 99 mg/kg/day.21 The World Health Organization (WHO) has established a maximum daily oral intake of benzyl alcohol for adults at 5 mg/kg/day, but no maximum IV dose has been established. For infants and children, WHO has not established a safe oral or IV maximum dose.21 Based on the reported toxicity of benzyl alcohol in preterm neonates and the high frequency of use of heparin flushes in critically ill neonates, only preservative-free normal saline and heparin flushes should be used in neonates. In addition, pharmacists should be vigilant in monitoring the amount of benzyl alcohol administered to neonates through other drugs such as dexamethasone, lorazepam, midazolam, and phenobarbital.
Propylene Glycol
Propylene glycol is commonly used as a solubilizer in topical, oral, and injectable medications. Propylene glycol toxicity — characterized by hyperosmolality, lactic acidosis, hemolysis, cardiac arrhythmia and seizures — has been reported in infants and children following exposure from these medications. Since propylene glycol is eliminated by the kidneys as well as metabolized by alcohol dehydrogenase in the liver, neonates and especially preterm neonates are predisposed to toxicity because of immature kidney and liver function.
The WHO has established a maximum daily oral intake of propylene glycol for adults at 25 mg/kg/day. Again, no maximum dose has been established for infants and children. Studies have shown that toxicity may occur when the serum propylene glycol concentration exceeds 25 mg/dL.22 Therefore, it is important that pharmacists monitor the cumulative dose as well as adverse reactions from propylene glycol when drugs such as etomidate and lorazepam are used in pediatric patients, especially when given via continuous infusions.
Ethanol
Ethanol is used as a solvent and preservative in liquid medication formulations. Besides central nervous system symptoms, acute ethanol poisoning can result in hypoglycemia, convulsions, and death in pediatric patients. While it is unclear how ethanol affects a developing brain, the AAP recommends that no ethanol should be included in medications intended for children. If ethanol must be used, it should not be no more than 5% (v/v), and each dose should not result in a blood alcohol level of more than 25 mg/dL.23
Thimerosal
Thimerosal is used as a preservative in vaccines, biological agents, and drugs. Thimerosal is metabolized to ethylmercury and thiosalicylate. Exposure to large amounts of ethylmercury (1,000- to 1,000,000-fold greater than the amount in vaccines) has been associated with permanent neurologic damage and death. However, no data show that low-dose thimerosal exposure from vaccines has been associated with the same neurological outcomes.
Fetal exposure to methylmercury, on the other hand, has been associated with neurological symptoms including psychomotor retardation, seizures, and impaired vision or hearing in human offspring. Nonetheless, toxicity of methylmercury cannot be extrapolated to that of ethylmercury. In addition, ethylmercury has a much shorter biological half-life than methylmercury (7 days compared with 50 days), hence it is less likely to accumulate and cause harm.24
Despite these facts, based on the theoretical concern of neurotoxicity and as a conservative measure, the FDA has worked with vaccine manufacturers to reduce to trace amounts or eliminate thimerosal from all routine childhood vaccines as of 2001. In addition, numerous states such as California and Washington have enacted mercury-free vaccine legislation to mandate that pregnant women and children under 3 years of age should only receive thimerosal-free vaccines. Pharmacists should be aware that multidose influenza vaccines do contain thimerosal and hence should be avoided in pregnant women and children younger than 3 years of age.
Aluminum
Aluminum is a ubiquitous metal that is poorly absorbed through the skin, respiratory tract, or gastrointestinal tract. The small amount of aluminum absorbed is eliminated in the urine. Despite the body’s natural barrier to aluminum, iatrogenic aluminum toxicity has been reported when medications contaminated with aluminum were administered to patients intravenously. Aluminum leaches from glass containers of IV medications over time and may also be found as a contaminant in raw materials used to manufacture medications.
When an excessive amount of aluminum is administered intravenously over time, it can accumulate in bones, block uptake of calcium, and lead to fractures, osteomalacia, and bone pain. When aluminum accumulates in the CNS, it can result in neurotoxicity such as encephalopathy. It can also cause microcytic hypochromic anemia and cholestasis. Additives in parenteral nutrition such as potassium phosphate, sodium phosphate, calcium gluconate, multivitamins, and trace elements have been recognized as a major source of aluminum exposure in pediatric patients. Neonates, especially preterm neonates, have decreased renal function and hence are at higher risk for aluminum toxicity. Patients with impaired renal function and those who are on long-term parenteral nutrition are also at risk.
In July 2004, the FDA mandated that intake of aluminum should not exceed 5 mcg/kg/day. In addition, large-volume parenteral products cannot exceed a maximum of 25 mcg/L of aluminum, and small-volume parenteral products must list the maximum aluminum concentration at the expiration date on the product label.25 Pharmacists should be aware of these mandates and choose products with the lowest aluminum contents for preparation of parenteral nutrition to reduce exposure and prevent potential aluminum toxicity in their patients.
DOSAGE FORMS
Oral
Oral tablets and capsules are designed for adult patients. Commercially available strengths are usually not appropriate for pediatric patients younger than 10 to 12 years of age. In addition, children under 5 years old usually have difficulty swallowing a tablet or capsule. The ideal oral formulation for younger pediatric patients is oral liquid. Unfortunately, not every medication used in pediatric patients is available in an oral liquid formulation. Moreover, some liquid formulations contain inactive ingredients such as alcohol that is not suitable for young children. Finally, palatability of oral solutions can pose an administration challenge to caregivers.
To circumvent these challenges, a few practices are commonly used when administering medications to pediatric patients. The IV formulation of the same medication can be administered orally. It is important to use the same salt form of the IV medication as the oral medication since a different salt form of a drug may have different extent of absorption. Furthermore, if a prodrug or delayed-release preparation is used for the oral formulation to circumvent absorption issues, using the IV medication orally would not be desirable. For example, enalaprilat IV should not be administered orally since enalaprilat is poorly absorbed. The prodrug, enalapril, should be used instead to ensure adequate absorption. Finally, while using an injectable product orally may work in an acute care setting, that may not be a good option in the ambulatory care setting because most prescription drug plans do not cover injectable products.
Another option is to crush a tablet or open the contents of a capsule and mix it in a fixed volume of water. The appropriate dose can then be drawn up as an aliquot. This method should be used only if the medication is water soluble. Otherwise, dosing will be inaccurate and vary greatly between doses since it is dependent on the number of undissolved particles captured in the aliquot.
Lastly, an extemporaneous formulation can be prepared if there are published studies to support the stability of the formulation. In general, alterations to the published formulation are discouraged since such changes can affect the pH and other chemical properties of the ingredients, thus altering the stability of the formulation. Pharmacists and pharmacy technicians who prepare extemporaneous formulations should be trained on the proper technique as well as standards that should be followed. The U.S. Pharmacopeia General Chapter <795> and American Society of Health-System Pharmacists (ASHP) Technical Assistance Bulletin on Compounding Nonsterile Products in Pharmacies are references that should be reviewed prior to setting up the environment, equipment, and policies and procedures for preparing extemporaneous formulations.
Flavoring agents can be used to improve palatability of an oral liquid. However, addition of flavoring agents can affect the pH and other chemical properties of the oral liquid and thereby reduce its shelf life. Therefore, flavoring agents should be added to the measured dose of the medication immediately before its administration. Flavoring agents should not be added to the entire bottle of the elixir, solution, or suspension unless testing has been performed to confirm the overall stability of the formulation.
Injectables
The concentrations of commercially available injectable medications are often inappropriate for neonates and young infants. Medications such as fentanyl are too concentrated to enable accurate measurement of the dose in a neonate, thus requiring dilution (see Illustration 1). On the other hand, medication concentrations usually used in adults may be too dilute for neonates and will use up a significant percentage of the total daily fluid allowed to administer all daily medications (see Illustration 2). The dead-space volume in delivery systems such as the hub of a syringe or hub of a needle (about 0.05 mL) becomes critical when a small volume of medication is being administered. When drug in the dead space is administered inadvertently, it can lead to a significant overdose. On the contrary, if drug that is supposed to be administered is trapped in the dead space, it can lead to a significant underdose (see Illustration 3 and Illustration 4). To minimize fluid administered for medications and conserve fluid for nutrition in neonates, the administration rate of continuous IV infusions can be as low as 0.1 mL/hr. If an adult IV set with an average of 15–25 mL priming volume is used, it would take days before the drug would reach the patient. Hence it is important that microbore tubing (priming volume 0.6–1.2 mL) is used in neonates and small infants and that the tubing is primed with drugs when critical infusions are administered to ensure immediate onset of action. As a general rule, syringe pumps should be used to administer IV medications in pediatric population to ensure accurate rate and volume administered.26
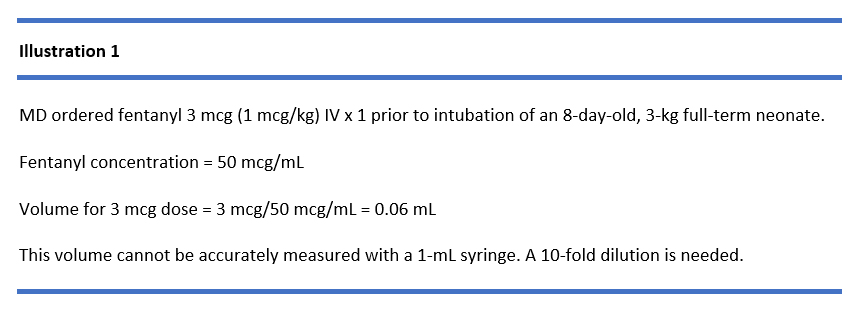
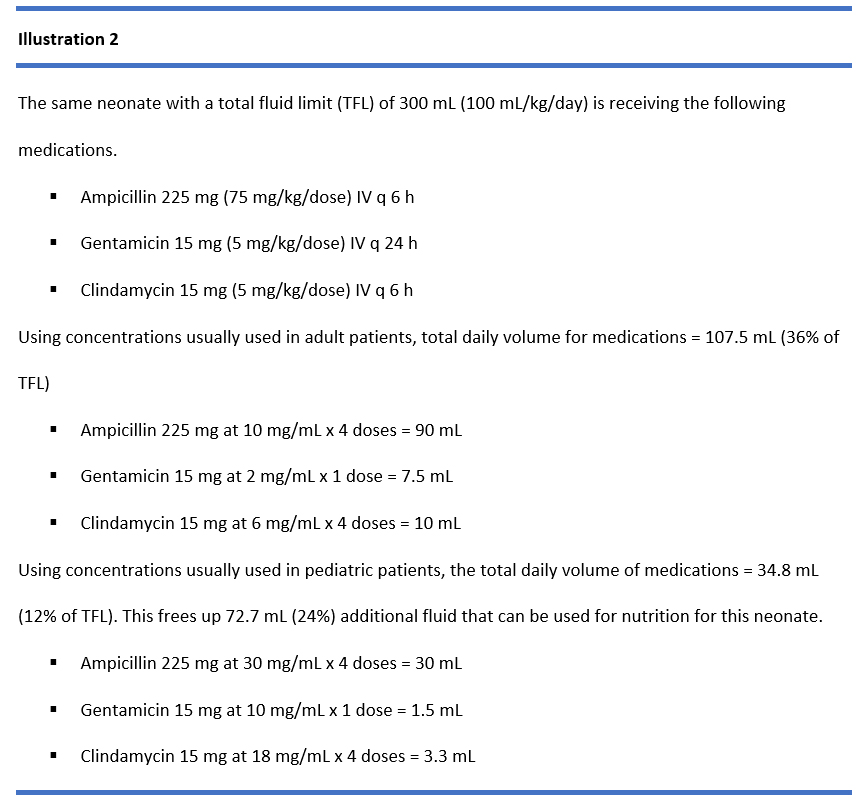
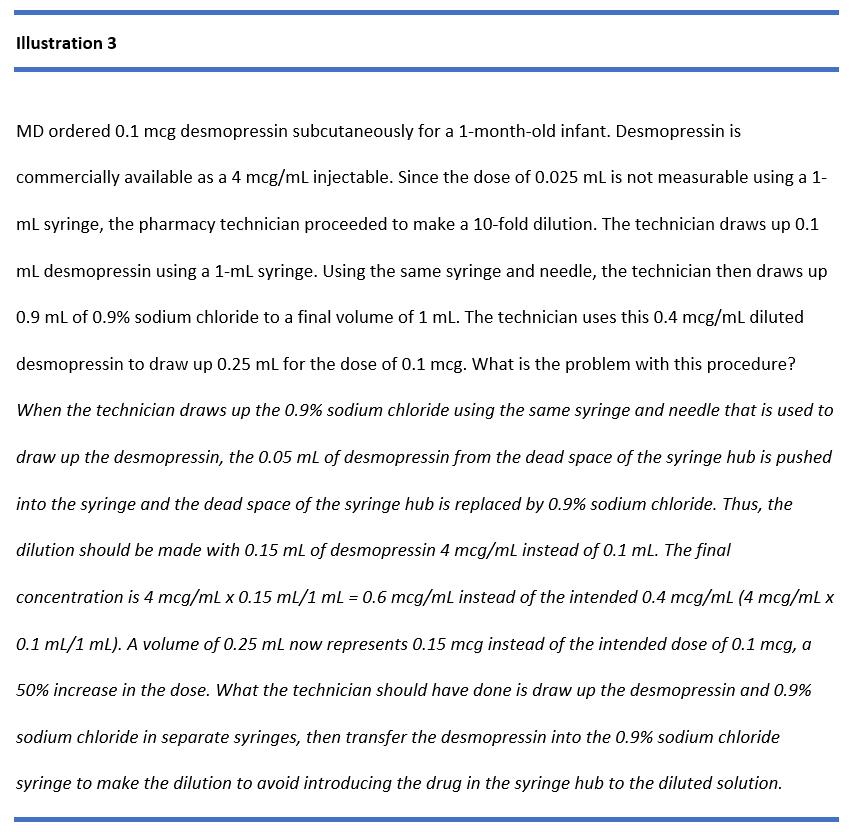
MEDICATION SAFETY
The different and changing pharmacokinetic parameters between pediatric patients at various ages and stages of maturational development coupled with the lack of published information or FDA-approved labeling regarding dosing, safety, efficacy, and clinical use of drugs in the pediatric population pose daily challenges to clinicians taking care of this patient population on proper use and dosing of medications in this patient population. Individualized doses based on the patient’s age, weight (mg/kg), body surface area (mg/m2), and clinical condition require accurate calculations for every dose administered. Common dosing errors include decimal misplacement (e.g., 0.1 vs. 0.01), incorrect unit conversion (e.g. dosing of infusion in mcg/kg/min and infusion concentration needs to be converted to mg/mL), misinterpretation of dosing guidelines (e.g., mg/kg/dose q 6 h vs. mg/kg/day divided q 6 h mix up), using pounds instead of kilograms for weight-based calculation, using mg/kg dosing in adult-size pediatric patients, resulting in the dose exceeding adult dose. The lack of commercially available dosage forms, concentrations, and unit-dose packaging appropriate for neonates, infants, and children often necessitates dilution of commercially available concentration, use of extemporaneous preparations, and patient-specific dose packaging. These manual activities are labor-intensive and time-consuming, and often require complex calculations, thus making them error prone.
Tending to the medication needs of this patient population also require precise dose measurement and appropriate drug-delivery systems. These complexities present huge challenges when devising safe and effective medication-use systems and processes to serve this patient population. Thus, it is not surprising that medication errors are 3 times more likely to occur in pediatric patients than in adults, making pediatrics the most vulnerable patient population in a hospital or health system.27
Culture of Safety
It is therefore essential to instill a culture of safety in every health professional involved in the care of pediatric patients and every setting in which they are seen. The following are some strategies to create a safe environment:
- Practice evidence-based medicine and ensure that the benefit always outweigh the risk
- Include a pediatric pharmacist on the multidisciplinary team caring for pediatric patients
- Ensure that policies and procedures are practical and being followed and discourage work arounds and short cuts
- Standardize and simplify equipment, supplies, and processes (e.g., standardize infusion concentrations, limiting strengths and concentrations of medications)
- Design jobs and working conditions for safety (e.g., minimize interruptions)
- Focus on improving reliability and safety in the medication use processes and avoid reliance on memory
- Leverage automation and technology such as computerized physician order entry, positive patient identification, smart pumps, robotics
- Report near misses and fix faulty systems, processes, and conditions
- Encourage all clinicians and patients/family to question orders when they are in doubt, and empower them to be persistent until they receive a reasonable/rational response (see Table 5 for unacceptable responses)
- Engage the patient and family as partners for safety check
- Ensure that children, parents, and other caregivers are educated on the child’s medication regimen, ideally by a pharmacist
Table 5. Unacceptable Responses to Queries About Medication Orders |
- That is what I want.
- That is what the attending physician or specialist recommended.
- The patient or parent says that’s what they take at home.
- I heard about this in a conference last week (journal reference not cited).
- This is a special case.
- The patient is very sick.
- That’s the protocol.
- The dose is from the patient’s old chart.
- It’s on the list of meds the patient gave me.
- We always give it that way.
|
Clinicians should be familiar with and put into practice the following national guidelines that promote safe medication use practices in pediatric patients:
- Pediatric Pharmacy Association (formerly Pediatric Pharmacy Advocacy Group [PPAG]) guidelines for preventing medication errors in pediatrics28
- ASHP-PPAG guidelines for providing pediatric pharmacy services in hospitals and health systems29
- ASHP Standardize 4 Safety Initiatives30
- Institute for Safe Medication Practice (ISMP)–targeted medication safety best practices for hospitals 2020–2021 that are especially important to pediatric patients (see Table 6)31
Table 6. ISMP Targeted Medication Safety Best Practices for Hospitals 2020–2021 Especially Important to Pediatric Patients |
Best Practice 1: Dispense vinCRIStine and other vinca alkaloids in a minibag of a compatible solution and not in a syringe. |
Best Practice 3: Weigh each patient as soon as possible on admission and during each appropriate outpatient or emergency department encounter. Avoid the use of a stated, estimated, or historical weight. Measure and document patient weights in metric units only. |
Best Practice 4: Ensure that all oral liquid medications that are not commercially available in unit dose packaging are dispensed by the pharmacy in an oral syringe or an enteral syringe that meets the International Organization for Standardization (ISO) 80369 standard, such as ENFit. |
Best Practice 5: Purchase oral liquid dosing devices (oral syringes/cups/droppers) that display only the metric scale. |
Best Practice 8: a) Administer medication infusions via a programmable infusion pump utilizing dose error-reduction systems. b) Maintain a 95% or greater compliance rate for the use of dose error-reduction systems. c) Monitor compliance with use of smart pump dose error-reduction systems on a monthly basis. d) If your organization allows for the administration of an intravenous bolus or a loading dose from a continuous medication infusion, use a smart pump that allows programming of the bolus (or loading dose) and continuous infusion rate with separate limits for each. |
Best Practice 11: When compounding sterile preparations, perform an independent verification to ensure that the proper ingredients (medications and diluents) are added, including confirmation of the proper amount (volume) of each ingredient prior to its addition to the final container. |
Best Practice 13: Eliminate injectable promethazine from the formulary. |
Best Practice 16: a) Limit the variety of medications that can be removed from an automated dispensing cabinet (ADC) using the override function. b) Require a medication order (e.g., electronic, written, telephone, verbal) prior to removing any medication from an ADC, including those removed using the override function. c) Monitor ADC overrides to verify appropriateness, transcription of orders, and documentation of administration. d) Periodically review for appropriateness the list of medications available using the override function. |
CONCLUSION
While not all pharmacists are pediatric specialists, all pharmacists should have a basic understanding that maturation impacts pharmacokinetics of medications in the different age group in pediatrics. All pharmacists also should be aware of the complexity and challenges when preparing and administering medications to pediatric patients. Armed with this fundamental information, all pharmacists are positioned to help safeguard the medication use process and protect this very vulnerable patient population.
References
- Benavides S, Nahata MC, ed. Pediatric Pharmacotherapy. Lenexa, KS: American College of Clinical Pharmacy; 2013: 18–33.
- Kearns GL, Abdel-Rahman SM, Alander SW, et al. Developmental pharmacology — drug disposition, action, and therapy in infants and children. N Engl J Med. 2003;349:1157–1167.
- Lim SY, Pettit RS. Pharmacokinetic considerations in pediatric pharmacotherapy. Am J Health-Syst Pharm. 2019;76:1472–1480.
- S. Food and Drug Administration. Should you put sunscreen on infants? Not usually. Updated July 6, 2016. Accessed July 20, 2020. https://www.fda.gov/consumers/consumer-updates/should-you-put-sunscreen-infants-not-usually
- Nieman LK. Consequences of systemic absorption of topical glucocorticoids. J Am Acad Dermatol. 2011;65(1):250–252.
- Begg EJ, Barclay ML. Aminoglycosides—50 years on. Br J Clin Pharmacol. 1995;39:597–603.
- Polymixin B sulfate. Micromedex® (electronic version). IBM Watson Health. Accessed July 20, 2020. https://www-micromedexsolutions-com.ucsf.idm.oclc.org/.
- Micromedex® (electronic version). IBM Watson Health. Accessed July 20, 2020. https://www-micromedexsolutions-com.ucsf.idm.oclc.org/
- Sathiyamurthy S, Banerjee J, Godambe Antiseptic use in the neonatal intensive care unit — a dilemma in clinical practice: an evidence based review. World J Clin Pediatr. 2016;5(2):159–171.
- Lexicomp® Online. Pediatric and Neonatal Lexi-Drugs Online. Accessed July 20, 2020. http://www.crlonline.com.ucsf.idm.oclc.org/lco/action/home
- O’Hara K, Wright IMR, Schneider JJ, et al. Pharmacokinetics in neonatal prescribing: evidence base, paradigms and the future. Br J Clin Pharmacol. 2015;80:1281–1288.
- Quigley EMM. Cisapride: what can we learn from the rise and fall of a prokinetic? J Digest Dis. 2011;12(3):147–156.
- Bory C, Baltassat P, Porthault M, et al. Metabolism of theophylline newborn infants. J Pediatr. 1979; 94:988–993.
- Miller RP, Roberts RJ, Fischer LJ. Acetaminophen elimination kinetics in neonates, children, and adults. Clin Pharmacol Ther. 1976;19:284–294.
- Muhari-Stark E, Burckart GJ. Glomerular filtration rate estimation formulas for pediatric and neonatal use. J Pediatr Pharmacol Ther. 2018;23:424–431.
- Micromedex® (electronic version). IBM Watson Health. Accessed July 20, 2020. https://www.ibm.com/watson-health
- Micromedex® (electronic version). IBM Watson Health. Accessed July 20, 2020. https://www-micromedexsolutions-com.ucsf.idm.oclc.org/
- Micromedex® (electronic version). IBM Watson Health, Greenwood Village, CO. Accessed July 20, 2020. https://www-micromedexsolutions-com.ucsf.idm.oclc.org/
- Brown WJ, Buist NRM, Gipson NTC, et al. Fatal benzyl alcohol poisoning in a neonatal intensive care unit. 1982;1:1250.
- Gershanik J, Boecler B, Ensley H, et al. The gasping syndrome and benzyl alcohol poisoning. N Engl J Med. 1982;307:1384–1388.
- Akinmboni TO, Davis NL, Falck AJ, et al. Excipient exposure in very low birth weight preterm neonates. J Perinatol. 2018;38:169–174.
- Lim TY, Poole RL, Pageler NM. Propylene glycol toxicity in children. J Pediatr Pharmacol Ther. 2014;19:277–282.
- American Academy of Pediatrics Committee on Drugs. Ethanol in liquid preparations intended for children. 1984;73:405–407.
- Offit PA, Jew RK. Addressing parents’ concerns: Do vaccines contain harmful preservatives, adjuvants, additives, or residuals? 2003;112:1394–1401.
- Poole RL, Pieroni KP, Gaskari S, et al. Aluminum in pediatric parenteral nutrition products measured versus labeled content. J Pediatr Pharmacol Ther. 2011;16(2):92–97.
- Jew RK, Gordin P, Lengetti E. Clinical implications of intravenous drug administration. Crit Care Nurse. 1997;17:62–70.
- Kaushal R, Bates DW, Landrigan C, et al. Medication errors and adverse drug events in pediatric inpatients. 2001;285:2114–2120.
- Levine SR, Cohen MR, Blanchard NR, et al. Guidelines for preventing medication errors in pediatrics. J Pediatr Pharmacol Ther. 2001;6:427–443.
- Eiland LS, Benner K, Gumpper KF, et al. ASHP-PPAG guidelines for providing pediatric pharmacy services in hospitals and health systems. Am J Hosp Pharm. 2018;75:1151–1165.
- American Society of Health-System Pharmacists. Standardize 4 Safety. Accessed July 20, 2020. https://www.ashp.org/Pharmacy-Practice/Standardize-4-Safety-Initiative
- ISMP Targeted Medication Safety Best Practices for Hospitals 2020–2021. Institute for Safe Medication Practices. Accessed July 20, 2020. https://www.ismp.org/sites/default/files/attachments/2020-02/2020-2021%20TMSBP-%20FINAL_1.pdf
Back to Top